Wettability and Adhesion Problems for Polypropylene (PP) Products
When applying coatings, inks, adhesives, and other materials to a surface, controlling the surface properties becomes critical. In particular, the wettability – the ability of the process liquid to spread and adhere to the solid surface – needs to be controlled. As more products rely on plastics, from medical devices to automobile bumpers, this concept has grown more important since plastics generally exhibit poor wettability for many liquids. This is due to the low surface free energy of many plastics. For example, polypropylene (PP), a widely used polymer due to its low cost, machinability, good chemical resistance and mechanical properties, is naturally hydrophobic, causing many water-based liquids to poorly wet the material.
Improving Wettability: Chemical Adhesion Promoter vs Plasma Treatment
To improve wettability, chemical adhesion promoters have been used, but cost and environmental concerns due to solvents such as toluene have prompted industry to seek alternatives such as flame [1] and plasma [2] treatment. Plasma treatment, in which the surface is placed in a chamber under vacuum and bombarded by ionized gas, is a safe and effective technique for increasing the wettability without harmful gases or high temperatures, and complex geometries can be easily treated. In the case of polymers like PP, wettability is enhanced by the formation of hydrophilic functional groups such as carbonyl, carboxyl, and hydroxyl at the surface [3].
Attention Theta Flex Optical Tensiometer Contact Angle Measurements
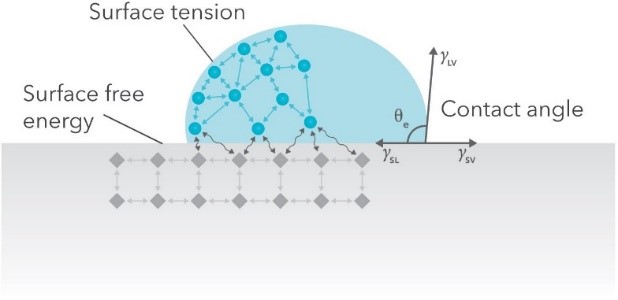
Here the surface properties of polypropylene are modified using plasma treatment. A Glow Plasma System is used to expose polypropylene samples to air plasma for different treatment times. Immediately after treatment, an Attention Theta Flex Optical Tensiometer is used to measure contact angles of several probe liquids on the treated surfaces. Using the contact angle data, the surface free energy, including changes to polar and dispersive surface energy components, is determined. The results highlight the complex dependence on treatment time as competitive surface reactions occur.
Adhesive Forces and Surface Tension
When a drop of liquid rests on a solid surface, molecules at the air-liquid and solid-liquid surfaces experience strong cohesive forces with their liquid neighbors and weaker adhesive forces with neighboring gas and solid molecules. The result is a surface tension (mN/m) that behaves similar to an elastic membrane. The same phenomenon occurs at the solid-gas surface. Each of these three surfaces have their own surface tensions: liquid-gas (γLV), solid-liquid (γSL), and solid-gas (γSV). Alternatively, γ can be interpreted as the energy required to form new surface area. In this view, γ takes the name surface free energy and can have the equivalent units mJ/m2.
At the three-phase contact line where the liquid, gas, and solid meet, each of these surface tensions are balanced by the contact angle θ made between the drop and the solid (Fig. 1). This balance is described by the Young equation [4],
The visual simplicity of Eq. 1 is deceptive. First, the equation assumes a smooth homogeneous surface, a pure liquid, and the entire system is in a thermodynamic equilibrium. Second, each surface tension can be considered the sum of a number of components representing different intermolecular forces: γLV=γLV^d+γLV^p+γLV^h+γLV^i+γLV^ab+γ^o where d, p, h, i, and ab respectively represent the dispersion, polar, hydrogen-bonding, induction, and acid-base interactions. The γ^o represents additional interactions. One of the more widely used approaches is to consider only the dispersive and polar components [5]. Using a geometric mean, the energy difference for a liquid molecule to reside at the surface becomes
A similar expression for the energy difference for a solid molecule to reside at the surface can also be found. Summing these energy differences for both a liquid and solid molecule residing at the surface gives the total energy required to form a new surface,
After combining Eq. 3 with Eq. 1, the extended Fowkes or OWRK equation is found [5]:
Equation 4 is used to find two unknowns, γSV^d and γSV^p, and thus contact angle measurements with at least two liquids with known γLV^d and γLV^p are needed. One liquid should be dominantly polar (e.g. water or glycerol) and one liquid should be dispersive (e.g. diiodomethane). Additional probe liquids with comparable polar and dispersive components such as ethylene glycol can be used to improve modeling. Afterward the total surface free energy of the solid-gas surface is γSV^tot=γSV^d+γSV^p.
Wettability and Surface Free Energy Experimentation: Plasma Treated vs Untreated Polypropylene (PP)
Squares of polypropylene 1×1 cm in size were cut from a single sheet of smooth untreated polypropylene (McMaster-Carr). The squares were washed with ethanol followed by DI water and dried with N2. Three probe liquids were used: ultrapure water (>18.2 MΩ⋅cm) (Millipore), 99% diiodomethane (Oakwood Chemical), and 99% ethylene glycol (Oakwood Chemical). The air-liquid surface tensions and dispersive and polar components of each probe liquid from ref. [6] are summarized in Table 1.
Polypropylene samples were treated with air plasma in the Glow Plasma System (Glow Research, Tempe, AZ) for durations ranging from 1 to 120 s. The plasma system operated at 50 W, 100 kHz, and the chamber pressure was 1.0 torr. After treatment the contact angles of each probe liquid were measured on the squares using an Attension Theta Flex Optical Tensiometer (Nanoscience Instruments, Phoenix, AZ). Each measurement occurred approximately 2 min immediately after the plasma treatment finished. Drop volumes were fixed at 2 μl. For each measurement, images were recorded for 1 s at 15 frame/s, and the contact angle was taken as the mean value from all 15 images. Experiments were performed in duplicate for each treatment time considered. Using the OneAttension software, the contact angles were used to determine the surface free energy of the polypropylene using the OWRK method.
Contact Angle Measurement Results with Attention Theta Flex Optical Tensiometer
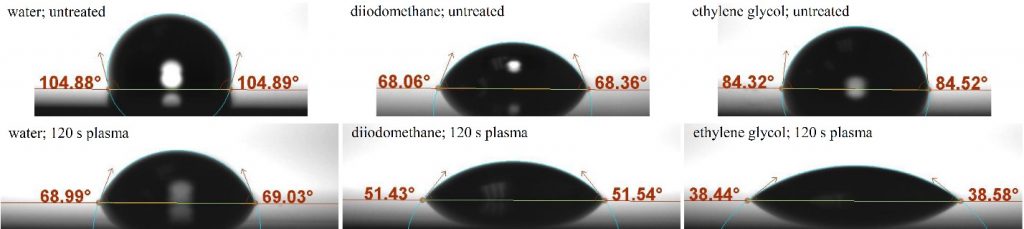
Figure 2 shows example sessile drop images with overlays from the OneAttension software indicating the drop profile fit (blue line) and measured contact angle. On untreated PP (top row of Fig. 2), the water contact angle clearly indicates a hydrophobic surface. After 120 s of exposure to plasma (bottom row), contact angles for each liquid decrease with the largest changes coming from water and ethylene glycol.
The mean contact angles for each probe liquid are plotted versus plasma treatment time in Fig. 3A. A drastic decrease in contact angles is observed in the first several seconds to 64°, but then the water contact angle increases to a local maximum at 15 s. The diiodomethane contact angle gradually increases for treatment times larger than 4 s, while the ethylene glycol contact angles remain roughly constant for the same treatment times. After 1 min the water contact angle approaches 69° while the diiodomethane approaches 58° and ethylene glycol approaches 42°.
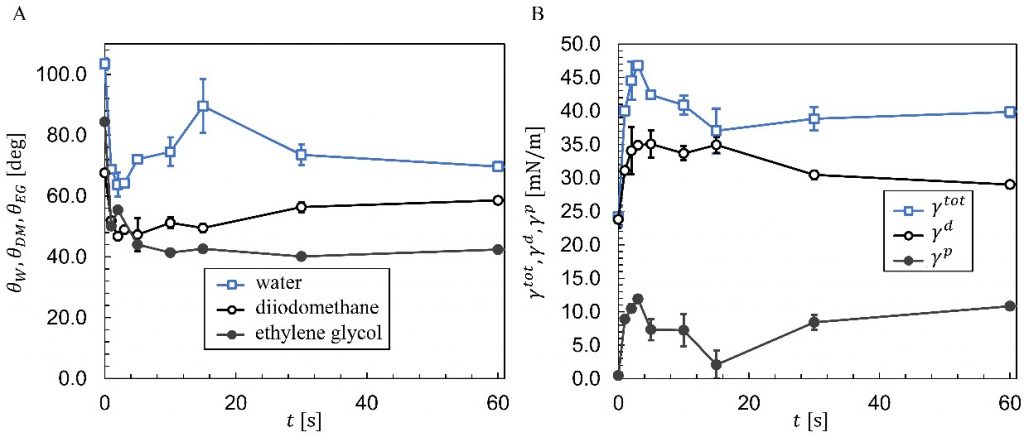
Surface Free Energy (SFE) Results Comparing Plasma Treatment and Untreated Polypropylene (PP)
Using contact angles in Fig. 3A, the OWRK method (Eq. 4) was used to determine the surface free energy (γ^tot) of the PP-air surface as well as the polar (γ^p) and dispersive (γ^d) components in Fig. 3B. This we done automatically in the OneAttension software. Initially γ^tot=24.2 mJ/m2 but then increases to 46.8 mJ/m2 after 3 s of treatment. The surface free energy then decreases to a local minimum at 15 s and afterwards increases, approaching 40 mJ/m2.
The dispersive and especially polar components are also quite dependent on treatment time. Initially γ^p~0 as the PP surface free energy is almost entirely dispersive. After only seconds of plasma exposure, γ^p increase up to 12 mJ/m2 while γ^d also increases to about 35 mJ/2. At 15 s though, the polar component drops significantly, corresponding to the drop in γ^tot and reduction in hydrophilicity in Fig. 3A. For treatment times greater than 15 s, γ^p gradually increases while γ^d decreases slightly. The dynamic nature of surface free energy and water contact angles agree with similar observations of plasma treated PVC [7] and PDMS [8].
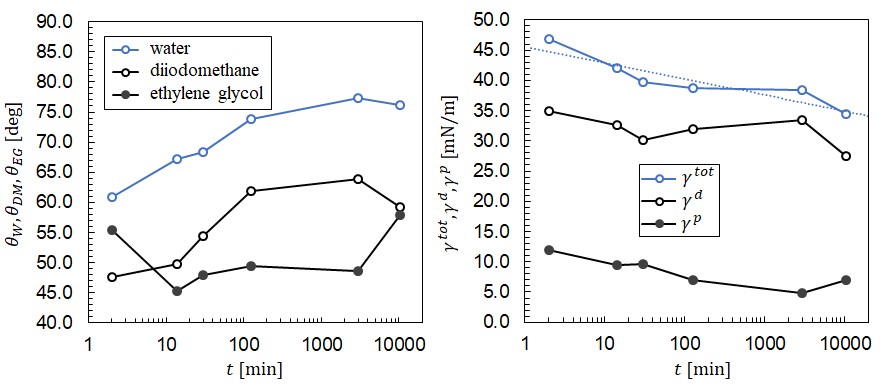
The effect of degradation of the enhanced wettability with time after initial exposure was also investigated in Fig. 4. Here, 6 samples of PP were simultaneously exposed to plasma for 3 s and then placed in a Petri dish in atmospheric conditions. One sample was selected for a SFE measurement at 2 min, 15 min, 30 min, 2 hr, 2 days, and 7 days. The resulting contact angles are shown in Fig. 4A on the semilog plot. The water contact angle quickly increases from 61 to 74 in the first two hours. After two hours, the water contact angle increases slightly over the course of a week but remains hydrophilic. In Fig. 4B the resulting reduction in SFE is shown versus time. The SFE decays roughly logarithmically with time with the form γ^tot=C1 lnt+C2 which is shown as the dotted line in Fig. 4B. The constants corresponding to this best fit are C1=-1.16 mJ/m2 and C2=45.6 mJ/m2 with t measured in min.
Improving Wettability of polypropylene (PP) by Plasma Treatment
The wettability of polypropylene (PP), a naturally hydrophobic material, was significantly improved using plasma treatment with the Glow Plasma System. With an Attension Theta Flex Optical Tensiometer, the contact angles of three probe liquids – water, diiodomethane, and ethylene glycol – were measured on PP after different plasma treatment times.
While the water contact angle on untreated PP is >100°, only 3 s exposure to the plasma dropped the contact angle to 64° and raised the surface free energy from 24.2 to 46.8 mJ/m2. However, increased treatment time subsequently reduced the surface free energy and wettability, with a local minimum in surface energy at 15 s. For treatment times >15 s, wettability gradually improved and surface free energy increased.
This time-dependency has been observed for plasma treatment of other polymers such as PVC [7] and PDMS [8]. The significant increase in PP polarity (γ^p) for short treatment times suggests efficient oxidation and formation of carboxylic, carbonyl, hydroxyl, and other hydrophilic functional groups. For treatment times between 5-15 s, however, competition between oxidation and subsequent reorientation, cross-linking and destruction of surface species [7] tends reduce the PP polarity, decrease surface free energy and decrease wettability. For the system used here, the greatest improvement in PP wettability and increase in surface free energy is observed for treatment times from 3-5 s.
The value of the quantitative capabilities of the Attension Theta Flex Optical Tensiometer and accompanying OneAttension software is demonstrated by the ability to precisely determine the optimum plasma treatment time for PP using air-plasma, and provide physical insight into the underlying process. Additional investigation into the effect of chamber pressure and power can also be studied with this technique. For any process requiring adhesion of liquids to polymers, the Theta platform and OneAttension software provide an efficient and intuitive means to optimize a plasma treatment protocol.
References
- [1] A.P. Pijpers, R.J. Meier. Adhesion behavior of polypropylenes after flame treatment determined by XPS(ESCA) spectral analysis. J. Electon Spectrosc. Relat. Phenom. 121 (2001) 299-313.
- [2] F. Awaja, M. Gilbert, G. Kelly, B. Fox, P.J. Pigram. Adhesion of polymers. Prog. Polym. Sci. 34 (2009) 948-968,
- [3] O.-J. Kwon, S. Tang, S.-W. Myung, N. Lu, H.-S. Choi. Surface characteristics of polypropylene film treated by an atmospheric pressure plasma. Surf. Coat. Technol. 192 (2005) 1-10.
- [4] P.C Hiemenz, R. Rajagopalan. “Principles of Surface and Colloids Chemistry.” Taylor & Francis Group, Boca Raton, FL, 1997.
- [5] D.K. Owens, R.C. Wendt. Estimation of the surface free energy of polymers. J. Appl. Polym. Sci. 13 (1969) 1741-1747.
- [6] W. Wu, R.F. Giese, Jr., C.J. van Oss. Evaluation of the Lifshitz-van der Waals/acid-base approach to determine surface tension components. Langmuir 11 (1995) 379-382.
- [7] H. Kaczmarek, J. Kowalonek, A. Szalla, A. Sionkowska. Surface modification of thin polymeric films by air-plasma or UV-irradiation. Surf. Sci. 507-508 (2002) 883-888.
- [8] S. Bhattacharya, A. Datta, J.M. Berg, S. Gangopadhyay. Studies on surface wettability of poly(dimethyl) siloxane (PDMS) and glass under oxygen-plasma treatment and correlation with bond strength. J. Microelectromech. S. 14 (2005) 590-597.